Baseball
Welcome and thanks for visiting...
Why Jump Testing Is Important For Baseball Players
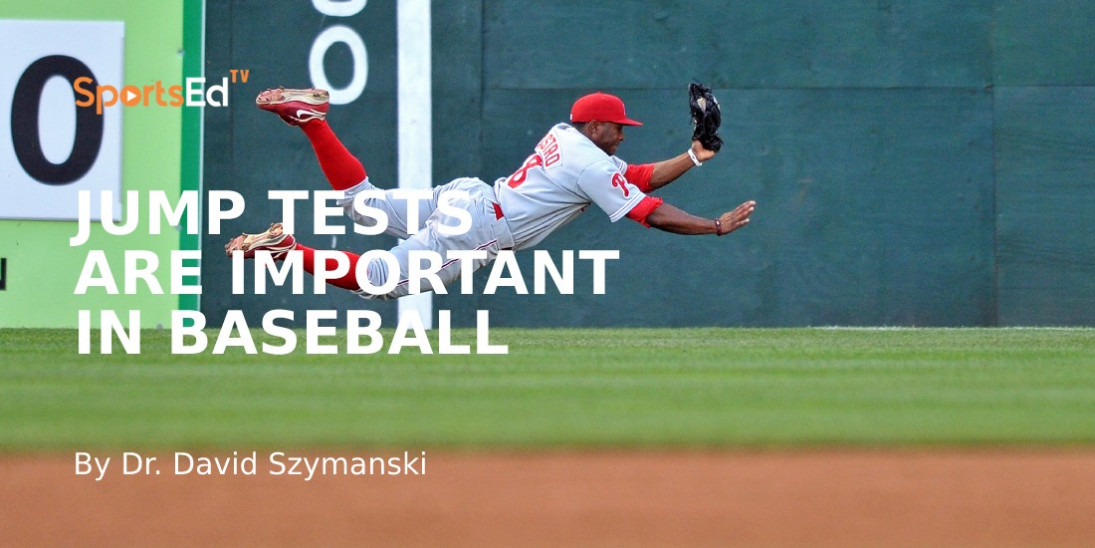
Power is an essential factor in achieving success in sport, and research by some has stated that power is equal to or more important than strength (2). Power is defined as force multiplied by velocity, or the product of speed and strength (1). Having an athlete perform a jump test, such as the vertical jump, demonstrates the athlete’s ability to produce power within a very short timeframe (< 0.3 sec) (6). Jump testing is relatively easy to perform, is low-cost, and is an effective method to assess explosiveness. Furthermore, various jump tests, such as vertical, broad, and lateral to medial jumps, have been demonstrated to be valid, reliable, and objective (1). Common vertical jump tests include bilateral and unilateral countermovement jumping while common horizontal jump tests include bilateral broad (standing long) jumping in the sagittal plane and unilateral lateral to medial jumping in the frontal plane. All of these tests can identify athletes with power deficiencies in specific planes.
The vertical jump test is a great tool for baseball players
Peak power, ground reaction forces (GRFs), and rate of force development (RFD) can be calculated from jumps off force plates. These variables have been suggested to be potentially more relevant to sport performance than simply jump height or distance (3,4,5). If force plates can be used while a pitcher throws or a batter hits, valuable information (peak power, GRFs, and RFD) can be collected about how the athlete applies forces in terms of the time at which force is applied and body mass relationships to power while performing a game-related task. If jump data (height, distance, or forces produced) and skilled performance data (pitch velocity, bat velocity, ball exit velocity) are compared and correlated, a coach could learn about the differences between individual players on a team as well as which variables have a relationship to game performance. If data is collected on high school, college, and professional baseball players, normative data tables can be created. These tables will allow for athlete comparisons at various ages and competitive levels that can possibly predict future talent. Additionally, this type of information can provide insight related to fatigue and potential injuries (4,7).
What Does Research Tell Us?
Recently in our labs, we completed research that looked at the relationship between various jump tests and their influence on multi-directional GRFs in baseball pitchers. Nineteen Division I collegiate baseball pitchers (age = 19.9 ± 1.5 yr, height = 1.86 ± 0.06 m, body mass = 90.7 ± 13. 8 kg) performed bilateral (Figure 1) and unilateral (Figure 2) countermovement vertical jump tests from force plates while using a Vertec jump height device, and bilateral broad jump (Figure 3) and unilateral lateral to medial jump (Figure 4) tests without force plates. Additionally, pitchers threw five fastballs from the wind-up and stretch from a custom-made pitching mound with two embedded force plates 18.44 m (60 ft 6 in) from home plate (Figure 5). Median fastball velocity recorded from a Rapsodo pitching device was used for analysis.
Figure 1. Bilateral countermovement vertical jump.
Figure 2. Unilateral countermovement vertical jump.
Figure 3. Broad (standing long) jump.
Figure 4. Unilateral lateral to medial jump.
Figure 5. Pitching test layout.
X-axis = anterior posterior direction (directed towards home plate in the intended path of the throw, Y-axis = mediolateral direction (directed towards first base for a right-handed pitcher), Z-axis = vertical direction (directed vertically), FP1 = drive leg force plate, FP2 = stride leg force plate
After various statistical tests (t-tests, Pearson product-moment correlations, and repeated measures ANOVA) were run, the results were as follows:
- There was one moderately high statistically significant relationship
- Dominant (drive leg) unilateral countermovement jump height and GRFs in the mediolateral direction (toward first base for a right-handed pitcher) of the stride leg during the wind-up (r = 0.64).
- There were three moderate statistically significant relationships
- Bilateral countermovement jump height to GRFs in the medial lateral direction for the stride leg in the pitching wind-up (r = 0.48).
- Bilateral countermovement jump height to anterior posterior (toward home plate) GRFs for the drive leg during the stretch delivery (r = 0.54).
- Non-dominant (stride leg) unilateral countermovement jump height revealed a moderate relationship with the GRFs in the anterior posterior direction of the drive leg during the stretch delivery (r = 0.47).
- Pitcher’s non-dominant (stride) leg unilateral countermovement vertical jump was significantly greater than their dominant (drive) leg unilateral countermovement vertical jump for:
- Height
- Peak power
- Normalized peak power by body weight
- Bilateral index %, defined as 100 x (bilateral measurement)/(right + left unilateral measurement) minus 100, was -27.24 ± 4.63 between the drive and stride leg unilateral countermovement jump.
- This indicates that pitchers are single leg dominant.
- There were contralateral effects between jump performance and pitching GRFs.
- This indicates that there is a cross-education effect.
- GRFs within the wind-up and stretch were similar.
- Fastball velocity from the wind-up and stretch were associated moderately high (r = 0.65 and r = 0.69) with drive (dominant) leg GRFs for the anterior posterior direction (toward home plate).
How Should This Jump Testing Information Be Practically Applied?
- It is important to test both bilateral and unilateral countermovement jumps.
- Collegiate pitchers were significantly more powerful vertically with their stride leg than their drive leg.
- This finding could be due to the stride leg being required to absorb the propulsion forces from the drive leg as the pitcher’s stride foot contacts the ground and decelerates the forward momentum created from the explosive motion toward home plate.
- Therefore, it is recommended that a resistance training program incorporate eccentric/force absorption exercises in the sagittal plane, such as depth jumps, forward step lunge, dumbbell forward step lunge and reach, step-up, or Nordic hamstring curl exercises.
- It is also recommended to resistance train the drive leg in the sagittal plane with the same single-leg multi-joint exercises mentioned above as it did perform significantly lower when compared to the stride (non-dominant) leg countermovement jump for height, absolute peak power, and normalized peak power by body weight.
- Drive leg anterior posterior (toward home plate) forces were related to fastball velocity.
- Therefore, it is recommended to include frontal plane single-leg multi-joint exercises, such as lateral squat, lateral lunge, and lateral lunge with plate press, to increase the amount of force produced within the drive leg to maintain fastball velocity or to possibly increase fastball velocity.
- In order to have a complete resistance training program, it is recommended to train bilateral multi-joint lower body exercises in the sagittal plane, such as back squat, front squat, deadlift as well and incorporate various double and single-leg lower body plyometric exercises in multiple planes and intensities.
- Forces within both pitching deliveries were similar, so improving force in one delivery should carry over into the other.
NOTE: For more information on using an estimation of Peak Power equation, check out Strength Training for Baseball..
References
- Coburn, J. W. (2012). Measuring power. Strength and Conditioning Journal, 34(6), 25–28.
- Kraemer, W. J., & Looney, D. P. (2012). Underlying mechanisms and physiology of muscular power. Strength and Conditioning Journal, 34(6), 13–19.
- Laffaye, G., Wagner, P. P., & Tombleson, T. I. L. (2014). Countermovement jump height: Gender and sport-specific differences in the force-time variables. Journal of Strength and Conditioning Research, 28(4), 1096–1105.
- Mayberry, J., Mullen, S., & Murayama, S. (2020). What can a jump tell us about elbow injuries in professional baseball pitchers? American Journal of Sports Medicine, 48(5), 1220–1225.
- Miller, A., MacDougall, J., Tarnopolsky, M., & Sale, D. (1993). Gender differences in strength and muscle fiber characteristics. European Journal of Applied Physiology and Occupational Physiology, 66(3), 254–262.
- Turner, A. N., Comfort, P., McMahon, J., Bishop, C., Chavda, S., Read, P., Mundy, P., & Lake, J. (2020). Developing powerful athletes, Part 1. Strength & Conditioning Journal, 42(3), 30–39.
- van Trigt, B., Leenen, T. (AJR), Hoozemans, M. (MJM), van der Helm, F. (FCT), & Veeger, D. (HEJ). (2020). Are UCL injuries a matter of bad luck? The role of variability and fatigue quantified. Proceedings, 49, 107.